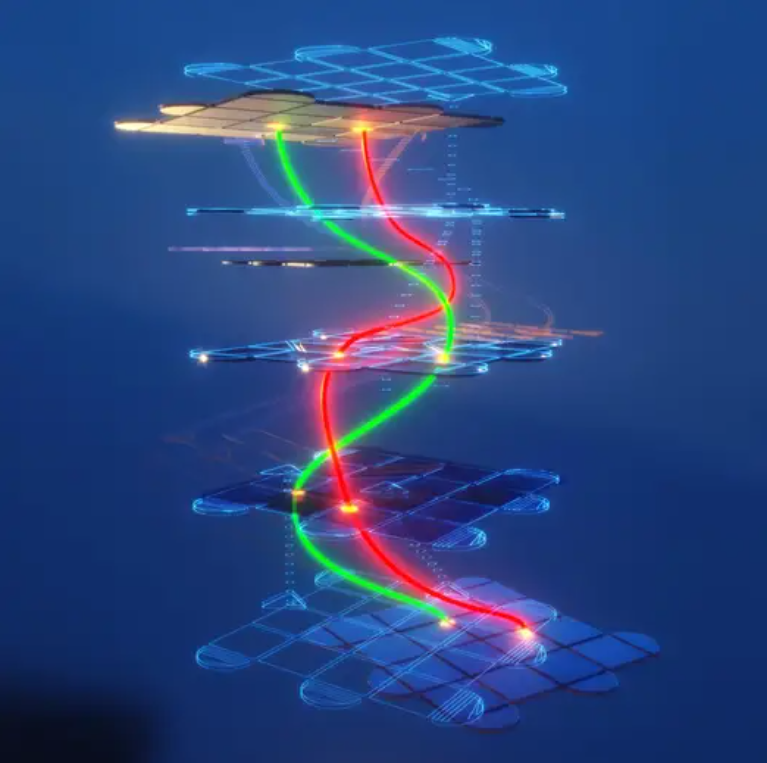
Google Quantum AI has made a groundbreaking observation of non-Abelian anyons, particles that can exhibit any intermediate statistics between the well-known fermions and bosons. This breakthrough has the potential to transform quantum computing by significantly enhancing its resistance to noise. The term “anyon” was coined by Nobel laureate physicist Frank Wilczek in the early 1980s while studying Abelian anyons. He combined “any” with the particle suffix “-on” to emphasize the range of statistics these particles can exhibit.
Fermions are elementary particles with half-integer spin, such as quarks and leptons (electrons, muons, tauons, as well as their corresponding neutrinos), and their wave functions are anti-symmetrical under the exchange of identical particles. Examples of bosons, which have integer spin and symmetrical wave functions under particle exchange, include the Higgs boson and the gauge bosons: photons, W- and Z bosons, and gluons. In contrast, anyons obey fractional quantum statistics and possess more exotic properties that can just exist in two-dimensional systems.
The history of anyons dates back to Nobel laureate Robert Laughlin’s study of the fractional quantum Hall effect, a phenomenon observed in two-dimensional electron systems subjected to strong magnetic fields. In 1983, he proposed a wave function to describe the ground state of these systems, which led to the understanding that the fractional quantum Hall effect involves quasiparticles with fractional charge and statistics. These quasiparticles can be considered as anyons in two-dimensional space.
Anyons can be categorized into two types: Abelian and non-Abelian. Abelian anyons obey Abelian (commutative) statistics, which were studied by Wilczek and Laughlin. Under particle exchange, they pick up a phase factor of e^i*theta, where theta is a scalar that is not just 0 as for bosons or pi as for fermions. Non-Abelian anyons, on the other hand, have more exotic properties: when exchanged, their quantum states change in a non-trivial way that depends on the order of the exchange, leading to a “memory” effect. Under particle exchange, their wavefunction picks up a phase factor of U=e^i*A with Hermitian matrix A that depends on the exchanged particles. As unitary matrices usually do not commute, it is this more-dimensional phase factor that explains the non-commutativity of non-Abelian anyons. This memory effect makes non-Abelian anyons particularly interesting for topological quantum computation. While the theoretical concept of non-Abelian anyons was already discussed around 1991, it was Alexei Kitaev who made the connection to fault-tolerant, topological quantum computing in a 1997 paper.
Microsoft, among other companies, has been working on harnessing non-Abelian anyons for topological quantum computing, focusing on a specific class called Majorana zero modes, which can be realized in hybrid semiconductor-superconductor systems. “Zero modes” in quantum mechanics refer to states that exist at the lowest energy level of a quantum system, also known as the ground state. Majorana fermions are a type of fermion that were first predicted by the Italian physicist Ettore Majorana in 1937. Their defining property is that they are their own antiparticles. This is unusual for fermions, which typically have distinct particles and antiparticles due to their charge (in contrast to a boson like the photon). While Majorana zero-modes have not been observed as elementary particles, they have found a home in the realm of condensed matter physics, specifically within certain “topological” materials. Here, they manifest as emergent collective behaviors of electrons, known as quasiparticles.
These quasiparticles, termed topological Majorana fermions, appear in the atomic structure of these materials. Intriguingly, they’re found in excited states, seemingly at odds with the “zero-mode” terminology which implies a ground state. The apparent contradiction can be resolved by understanding that Majorana zero modes are ground states within their own subsystem, the specific excitation they form. However, their presence indicates an excited state for the overall electron system, compared to a state with no Majorana zero modes. In other words, they are a ground state property of an excited electron system.
In a recent paper published in Nature on May 11, 2023, Google Quantum AI reported their first-ever observation of non-Abelian anyons using a superconducting quantum processor (see also article on arXiv from 19 Oct 2022). They demonstrated the potential use of these anyons in quantum computations, such as creating a Greenberger-Horne-Zeilinger (GHZ) entangled state by braiding non-Abelian anyons together.
This achievement complements another recent study published on May 9, 2023, by quantum computing company Quantinuum, which demonstrated non-Abelian braiding using a trapped-ion quantum processor. The Google team’s work shows that non-Abelian anyon physics can be realized on superconducting processors, aligning with Microsoft’s approach to quantum computing. This breakthrough has the potential to accelerate progress towards fault-tolerant topological quantum computing.
Leave a Reply